Downscale Finite Element Modeling of Aortic Valve Leaflets for In-Situ Estimation of Cell Level Mechanics
As in all tissues, mechanical forces in the aortic valve (AV) modulate the constituent cell population’s physiology and biosynthetic activity. While advances have been made toward the understanding of this complex multi-scale relationship, the specific role that and extracellular matrix (ECM) coupling plays on the mechanical response of the AV interstitial cell (AVIC) is poorly understood. The current work, building on our previous preliminary work [1], explores the impact of cellular contractile behavior on effective tissue level stiffness in an effort to gain insight into the complex mechanisms involved. By developing a computational tool to separate intrinsic cell mechanics from the ECM in-situ, we can obtain an accurate representation of AVIC behavior within the native valve connective tissue matrix microenvironment. Two computational finite element (FE) models were developed using COMSOL Multiphysics 4.3 that represent the tissue-level (macro-scale) and the cell level (micro-scale) of the aortic heart valve. These two models were coupled using a downscale technique that maps the deformation computed on the macro-scale to boundary conditions on layer-specific representative volume elements of the micro-scale simulation (Figure 1, A). The objective of the macro-scale model was to estimate the effective ECM stiffness from the moment-curvature relationship of AV leaflets using data from a previous study [2]. Briefly, a three point bending device (Figure 1, B) was used to characterize the flexural rigidity of porcine AV leaflets using Euler Bernoulli Beam theory. Transmural effects were examined by loading the leaflets both with and against the natural curvature of the leaflet. Testing was executed under conditions of basal tonus, hyper-contraction, and inhibited contraction to decouple cell-ECM coupling effects. From the resulting data, the macro-scale model was validated and the down-scale FE model was used to estimate the layer-specific tissue properties at varying states of cellular contraction and to probe the influence of cell-ECM stiffness on the overall macro-level mechanical properties. To further extend this initial multi-scale model to a more micro-anatomically accurate representation, both the AVIC ultrastructure and tissue microstructure were examined using transmission electron microscopy (TEM) and multi-photon microscopy (MPM) respectively. Biochemical and histological analysis of ECM components (collagen, elastin, glycosaminoglycan) and AVIC density was done to characterize transmural changes of tissue properties. These layer-specific structural properties such as ECM mass content, collagen fiber alignment and AVIC density and orientation were then implemented into the micro-scale FEM model. Using a homogeneous isotropic hyperelastic model to approximate the ECM response, we demonstrated that the current experimental data for cell stiffness using AFM of 50kPa is reasonably accurate, as in [1]. Moreover, we have determined that there are significant layer differences in effective cell stiffness and the relative coupling between the cells and the ECM. Currently, a structural hyperelastic constitutive model that more accurately represents the mechanical properties of the tissue is being implemented to further investigate these findings. This current modeling approach provides a sensitive method to estimate cell and ECM mechanical properties in situ from tissue level experimental measurements.
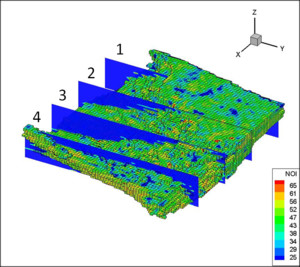
Téléchargement
- buchanan_presentation.pdf - 3.18MB
- buchanan_abstract.pdf - 0.32MB