Design Optimization for MRI-Compatible, Transparent PEDOT:PSS Neural Implants using Mesh Strategy
Magnetic Resonance Imaging (MRI) is a powerful tool for exploring brain function, offering detailed insights into its structure and physiology. Combining MRI technology with electrophysiological recording systems can enhance comprehension of brain functionality through synergistic effects. However, integrating neural implants with MRI technology poses challenges due to strong electromagnetic (EM) fields during MRI scans. MRI-compatible neural implants must enable detailed investigation of neural activities in real-time with high resolution without compromising patient safety or imaging quality.
In this work, we introduce a fully MRI-compatible monolayer pristine open-mesh PEDOT:PSS neural interface to address challenges encountered with traditional metal-based electrodes in the MRI environment, such as induced heat or imaging artifacts. PEDOT:PSS exhibits diamagnetic properties with lower electrical conductivity and negative magnetic susceptibility similar to human tissue. Furthermore, electromagnetic heating effects in brain tissue were simulated using COMSOL Multiphysics. The simulation setup involved a brain tissue phantom within an air medium, with electromagnetic wave-generating coils along each axis. The simulation integrated the Electromagnetic Waves module (frequency-domain) with the Bioheat Transfer module to model the thermal response of brain tissue to electromagnetic fields. An electrode within the brain tissue experienced electromagnetic heating, with heat transfer analyzed using the Electromagnetic Waves module (frequency-domain) coupled with the Heat Transfer in Solids module. The exchange of conduction heat between the electrode and brain tissue interfaces was examined until thermal equilibrium was reached.
The utilization of PEDOT:PSS electrodes presents a promising avenue for enhancing MRI compatibility compared to Pt electrodes. However, optimization of probe patterns holds potential for further performance enhancement. Leveraging photolithography, various open and closed designs were fabricated and compared against block designs. Closed contour loop area (CCLA) emerged as a pivotal parameter influencing induced current optimization. Open designs exhibited reduced CCLA compared to closed designs, offering scope for further improvement in mesh structure and trace intersections. COMSOL simulation facilitated mesh design optimization to minimize temperature rise and induced voltage/current, thereby reducing image artifacts.
From a design perspective, Open Mesh III demonstrated superior MRI compatibility compared to Open Mesh II and Open Mesh I, with a 46% lower electric field magnitude than Closed Mesh. Electric field variations were mirrored in temperature rise profiles across different structures, with optimized Open Mesh III showing a maximum peak temperature rise of 0.3 K, slightly higher than control tissue. Temperature rise increased with scale size and resonant frequency. Evaluation of MRI image artifacts proved challenging due to the complexity of phase and frequency encoding. Magnetic field gradient analysis offered a practical approach, reflecting induced changes in magnetic field disturbance. Closed mesh and block structures exhibited significantly higher magnetic field gradients compared to Open Mesh III, highlighting the latter's efficacy in reducing image distortion.
Overall, optimization of PEDOT:PSS electrode patterns, guided by COMSOL simulation, holds promise for enhancing MRI compatibility and analyzing the maximum temperature and eddy current induced in the neural probe. These findings underscore the importance of meticulous design refinement in minimizing image artifacts and advancing neural interface technology for robust neuroimaging applications.
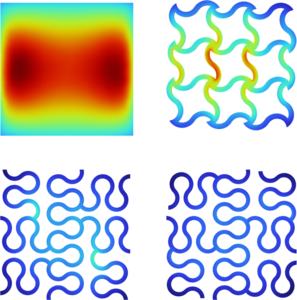